Absolute Rate Definition
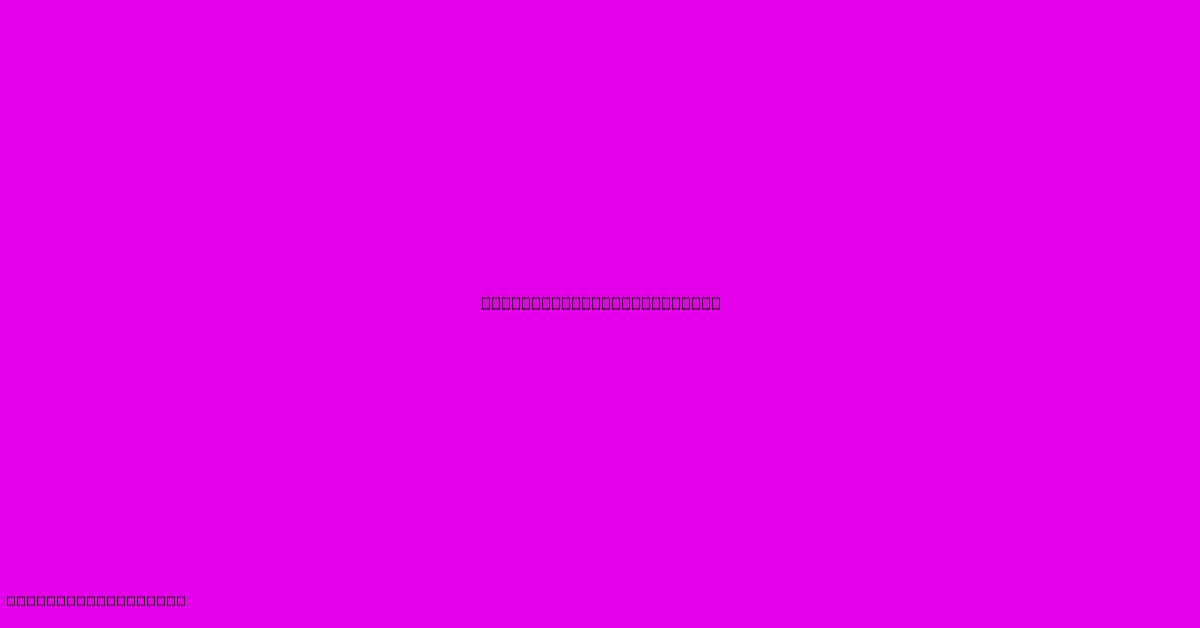
Discover more in-depth information on our site. Click the link below to dive deeper: Visit the Best Website meltwatermedia.ca. Make sure you don’t miss it!
Table of Contents
Unveiling Absolute Rate: A Deep Dive into Reaction Kinetics
Hook: How quickly do chemical reactions truly unfold? Understanding absolute reaction rates is crucial for predicting and controlling chemical processes across diverse fields.
Editor's Note: This comprehensive guide to absolute rate theory has been published today.
Relevance & Summary: Absolute rate theory, also known as transition state theory, provides a powerful framework for calculating reaction rates. This understanding is fundamental in chemical kinetics, impacting fields from catalysis and materials science to biochemistry and environmental chemistry. This guide will explore the core concepts, applications, and limitations of absolute rate theory. We will examine its mathematical underpinnings, focusing on activation energy, frequency factors, and the influence of temperature. The discussion includes exploring the Eyring equation, its derivation, and practical implications.
Analysis: The information presented here is synthesized from established textbooks and peer-reviewed research articles on chemical kinetics and physical chemistry. The analysis focuses on clearly explaining the theory and its practical applications, avoiding overly complex mathematical derivations while maintaining scientific rigor.
Key Takeaways:
- Absolute rate theory quantifies reaction rates by considering the transition state.
- The Eyring equation is central to calculating reaction rates.
- Activation energy and temperature significantly influence reaction rates.
- The theory has limitations, particularly for complex reactions.
- Understanding absolute rate theory is crucial for diverse scientific fields.
Absolute Rate Theory: A Microscopic Look at Reaction Rates
Absolute rate theory offers a powerful method to calculate the rate of a chemical reaction based on the properties of the reacting molecules and their transition state. Unlike other rate theories that focus solely on macroscopic observations, absolute rate theory delves into the microscopic details of the reaction process. This provides valuable insights into the factors governing reaction speeds, enabling predictions and manipulations of reaction kinetics.
Key Aspects of Absolute Rate Theory
Absolute rate theory hinges on several critical aspects:
- The Transition State: The theory postulates the existence of a transition state (also known as the activated complex), a high-energy, unstable intermediate structure formed during the conversion of reactants to products. This transition state represents the point of maximum potential energy along the reaction pathway.
- Activation Energy: The energy difference between the reactants and the transition state is the activation energy (Ea). This energy barrier must be overcome for the reaction to proceed. Reactions with lower activation energies tend to be faster.
- Frequency Factor (A): This factor represents the frequency with which reactant molecules approach the transition state with the correct orientation and sufficient energy.
- Temperature Dependence: The rate of a reaction is highly sensitive to temperature, with rate constants generally increasing exponentially with temperature. This temperature dependence is directly related to the activation energy.
The Eyring Equation: The Heart of Absolute Rate Theory
The central equation in absolute rate theory is the Eyring equation:
k = (k<sub>B</sub>T/h) * exp(-ΔG‡/RT)
where:
- k is the rate constant
- k<sub>B</sub> is the Boltzmann constant
- T is the absolute temperature
- h is Planck's constant
- ΔG‡ is the Gibbs free energy of activation
- R is the ideal gas constant
This equation directly links the rate constant to thermodynamic parameters of the transition state. The Gibbs free energy of activation (ΔG‡) encapsulates the enthalpy (ΔH‡) and entropy (ΔS‡) changes associated with reaching the transition state. The Eyring equation can also be expressed in terms of these parameters:
k = (k<sub>B</sub>T/h) * exp(ΔS‡/R) * exp(-ΔH‡/RT)
This form highlights the influence of both enthalpy and entropy on the reaction rate. A negative ΔH‡ indicates an exothermic process leading to faster reaction, while a positive ΔS‡ (more disorder) favors a faster reaction.
Applications of Absolute Rate Theory
Absolute rate theory finds wide applicability across numerous scientific disciplines:
- Catalysis: Understanding the activation energy and transition state structure is crucial for designing efficient catalysts that lower the activation energy barrier and increase reaction rates.
- Materials Science: The theory helps in predicting the rates of solid-state reactions, which are essential in the synthesis and processing of new materials.
- Biochemistry: Absolute rate theory plays a significant role in understanding enzyme kinetics, explaining the remarkable catalytic efficiency of enzymes. The theory helps in understanding the interaction between enzymes and substrates, along with the transition state.
- Environmental Chemistry: Understanding reaction rates is critical for predicting the fate and transport of pollutants in the environment. Absolute rate theory helps model degradation processes and chemical transformations.
Delving Deeper: Key Aspects of Absolute Rate Theory
Activation Energy and its Influence
Activation energy is the cornerstone of absolute rate theory. It represents the minimum energy required for reactant molecules to overcome the energy barrier and reach the transition state. A lower activation energy implies a faster reaction rate, as more molecules possess sufficient energy to surmount the barrier at a given temperature. The Arrhenius equation, although not directly derived from absolute rate theory, is closely related and provides a useful empirical relationship between the rate constant and activation energy:
k = A * exp(-Ea/RT)
where A is the pre-exponential factor (similar but not identical to the frequency factor in Eyring equation).
The Frequency Factor and its Significance
The frequency factor (or pre-exponential factor) represents the frequency of collisions between reactant molecules with the correct orientation and sufficient energy to reach the transition state. This factor incorporates both the frequency of collisions and the probability of successful collisions. A higher frequency factor leads to a faster reaction rate. The frequency factor is influenced by factors such as steric effects and molecular vibrations.
Temperature's Impact on Reaction Rates
Temperature plays a crucial role in influencing reaction rates, as predicted by both the Arrhenius and Eyring equations. An increase in temperature increases the average kinetic energy of the molecules, resulting in a higher proportion of molecules possessing sufficient energy to overcome the activation energy barrier. This leads to an exponential increase in the reaction rate. The temperature dependence is expressed explicitly through the exponential term in both equations.
Limitations of Absolute Rate Theory
While absolute rate theory offers a powerful framework, it has limitations:
- Assumption of Equilibrium: The theory assumes that the transition state is in equilibrium with the reactants. This assumption may break down for fast reactions or under non-equilibrium conditions.
- Simplified Model: The theory often employs simplified models for the transition state structure, neglecting factors such as tunneling and vibrational effects.
- Complex Reactions: Absolute rate theory becomes more challenging to apply to complex multi-step reactions.
Connecting the Dots: Absolute Rate Theory and its Practical Implications
Catalysis and its Enhancement of Reaction Rates
Catalysts significantly accelerate reaction rates by lowering the activation energy. By providing an alternative reaction pathway with a lower energy barrier, catalysts allow more reactant molecules to reach the transition state, thus increasing the reaction rate. Absolute rate theory helps in understanding how catalysts achieve this, providing insights into the design and optimization of catalysts.
Enzyme Kinetics and Biological Processes
In biological systems, enzymes act as highly specific and efficient catalysts. Absolute rate theory provides a framework for understanding enzyme kinetics, allowing for a detailed analysis of the interaction between enzymes and substrates. By examining the transition state of the enzyme-substrate complex, researchers can gain valuable insights into the catalytic mechanism.
Environmental Chemistry and Pollutant Degradation
The degradation of pollutants in the environment is often governed by chemical reaction rates. Absolute rate theory allows for modeling the rates of these reactions, helping predict the persistence of pollutants and evaluate strategies for remediation.
FAQ: Absolute Rate Theory
Introduction: This section addresses frequently asked questions about absolute rate theory.
Questions:
-
Q: What is the key difference between absolute rate theory and other rate theories? A: Absolute rate theory focuses on the microscopic properties of the transition state, while other theories, like collision theory, primarily rely on macroscopic observations.
-
Q: What are the limitations of absolute rate theory? A: The assumption of equilibrium between reactants and the transition state may not always hold true, and the theory simplifies the transition state structure.
-
Q: How does temperature affect reaction rates according to absolute rate theory? A: Increasing temperature increases the proportion of molecules possessing sufficient energy to overcome the activation barrier, leading to an exponential increase in the rate constant.
-
Q: What is the significance of the activation energy? A: The activation energy is the energy barrier that must be overcome for the reaction to proceed. Lower activation energies lead to faster reactions.
-
Q: How is absolute rate theory applied in catalysis? A: It helps in understanding how catalysts lower the activation energy, providing insights into the design of more effective catalysts.
-
Q: How is absolute rate theory used in biochemistry? A: It helps explain the remarkable catalytic efficiency of enzymes by providing a detailed analysis of the enzyme-substrate interaction and transition state.
Summary: Understanding absolute rate theory offers crucial insights into the factors governing reaction rates.
Tips for Understanding Absolute Rate Theory
Introduction: This section offers practical tips for effectively grasping the concepts of absolute rate theory.
Tips:
- Start with the Basics: Ensure a solid grasp of fundamental concepts like activation energy, Gibbs free energy, and equilibrium before delving into the theory.
- Visualize the Transition State: Develop a mental picture of the transition state as an intermediate structure representing the point of maximum potential energy.
- Focus on the Eyring Equation: Understand the derivation and implications of the Eyring equation, appreciating its relationship to thermodynamic parameters.
- Connect the Theory to Real-World Examples: Apply the theory to real-world scenarios, such as catalysis or enzyme kinetics, to reinforce your understanding.
- Practice Problem Solving: Work through examples and problems to solidify your understanding of the mathematical aspects of the theory.
- Explore Advanced Topics: Once comfortable with the basics, delve into advanced topics such as tunneling effects and the treatment of complex reactions.
Summary: By using these tips, you can build a robust understanding of absolute rate theory.
Summary of Absolute Rate Theory
This article provided a comprehensive exploration of absolute rate theory, detailing its key concepts, applications, and limitations. The theory provides a powerful framework for understanding and predicting chemical reaction rates at a molecular level. Its applicability spans various scientific disciplines, impacting advancements in catalysis, materials science, biochemistry, and environmental chemistry.
Closing Message: A thorough understanding of absolute rate theory is crucial for anyone working in fields where reaction kinetics are paramount. Continued research and development in this area promise further insights into the fundamental processes governing chemical reactions, paving the way for novel technologies and applications.
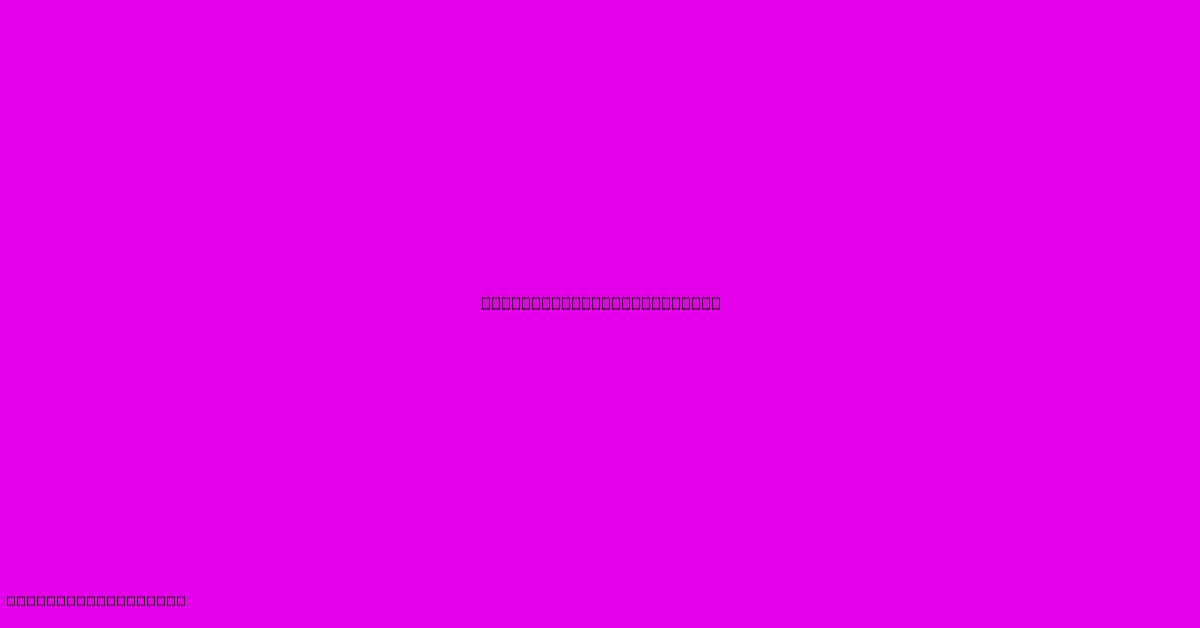
Thank you for taking the time to explore our website Absolute Rate Definition. We hope you find the information useful. Feel free to contact us for any questions, and don’t forget to bookmark us for future visits!
We truly appreciate your visit to explore more about Absolute Rate Definition. Let us know if you need further assistance. Be sure to bookmark this site and visit us again soon!
Featured Posts
-
What Is The Excess Return For The Portfolio Of Small Stocks
Jan 10, 2025
-
Assigned Risk Definition
Jan 10, 2025
-
Add On Interest Definition Formula Cost Vs Simple Interest
Jan 10, 2025
-
Revenue Agent Definition
Jan 10, 2025
-
Asset Accumulation Definition
Jan 10, 2025